Authors
K. Shu, Y. Tajima, R. Uozumi, N. Miyamoto, S. Shiraishi, T. Kobayashi, A. Ishida, K. Yamada, R. W. Gladen, T. Namba, S. Asai, K. Wada, I. Mochizuki, T. Hyodo, K. Ito, K. Michishio, B. E. O'Rourke, N. Oshima, K. Yoshioka
Abstract
When laser radiation is skilfully applied, atoms and molecules can be cooled1,2,3, allowing the precise measurements and control of quantum systems. This is essential for the fundamental studies of physics as well as practical applications such as precision spectroscopy4,5,6,7, ultracold gases with quantum statistical properties8,9,10 and quantum computing. In laser cooling, atoms are slowed to otherwise unattainable velocities through repeated cycles of laser photon absorption and spontaneous emission in random directions. Simple systems can serve as rigorous testing grounds for fundamental physics—one such case is the purely leptonic positronium11,12, an exotic atom comprising an electron and its antiparticle, the positron. Laser cooling of positronium, however, has hitherto remained unrealized. Here we demonstrate the one-dimensional laser cooling of positronium. An innovative laser system emitting a train of broadband pulses with successively increasing central frequencies was used to overcome major challenges posed by the short positronium lifetime and the effects of Doppler broadening and recoil. One-dimensional chirp cooling was used to cool a portion of the dilute positronium gas to a velocity distribution of approximately 1 K in 100 ns. A major advancement in the field of low-temperature fundamental physics of antimatter, this study on a purely leptonic system complements work on antihydrogen13, a hadron-containing exotic atom. The successful application of laser cooling to positronium affords unique opportunities to rigorously test bound-state quantum electrodynamics and to potentially realize Bose–Einstein condensation14,15,16,17,18 in this matter–antimatter system.
References
-
Chu, S. Nobel lecture: the manipulation of neutral particles. Rev. Mod. Phys. 70, 685–706 (1998).
-
Cohen-Tannoudji, C. N. Nobel lecture: manipulating atoms with photons. Rev. Mod. Phys. 70, 707–719 (1998).
-
Phillips, W. D. Nobel lecture: laser cooling and trapping of neutral atoms. Rev. Mod. Phys. 70, 721–741 (1998).
-
Bloch, I. Ultracold quantum gases in optical lattices. Nat. Phys. 1, 23–30 (2005).
-
Takamoto, M., Hong, F. L., Higashi, R. & Katori, H. An optical lattice clock. Nature 435, 321–324 (2005).
-
Andreev, V. et al. Improved limit on the electric dipole moment of the electron. Nature 562, 355–360 (2018).
-
Safronova, M. S. et al. Search for new physics with atoms and molecules. Rev. Mod. Phys. 90, 025008 (2018).
-
Cornell, E. A. & Wieman, C. E. Nobel lecture: Bose-Einstein condensation in a dilute gas, the first 70 years and some recent experiments. Rev. Mod. Phys. 74, 875–893 (2002).
-
Ketterle, W. Nobel lecture: when atoms behave as waves: Bose-Einstein condensation and the atom laser. Rev. Mod. Phys. 74, 1131–1151 (2002).
-
Regal, C. A., Greiner, M. & Jin, D. S. Observation of resonance condensation of fermionic atom pairs. Phys. Rev. Lett. 92, 040403 (2004).
-
Deutsch, M. Evidence for the formation of positronium in gases. Phys. Rev. 82, 455–456 (1951).
-
Cassidy, D. B. Experimental progress in positronium laser physics. Eur. Phys. J. D 72, 53 (2018).
-
Baker, C. J. et al. Laser cooling of antihydrogen atoms. Nature 592, 35–42 (2021).
-
Platzman, P. M. & Mills, A. P. Jr Possibilities for Bose condensation of positronium. Phys. Rev. B 49, 454–458 (1994).
-
Morandi, O., Hervieux, P. A. & Manfredi, G. Bose-Einstein-condensation dynamics with a quantum-kinetic approach. Phys. Rev. A 88, 023618 (2013).
-
Morandi, O., Hervieux, P. A. & Manfredi, G. Bose-Einstein condensation of positronium in silica pores. Phys. Rev. A 89, 033609 (2014).
-
Shu, K. et al. Study on cooling of positronium for Bose–Einstein condensation. J. Phys. B 49, 104001 (2016).
-
Mills, A. P. Positronium Bose-Einstein condensation in liquid 4He bubbles. Phys. Rev. A 100, 063615 (2019).
You May Also Like
These Related Stories
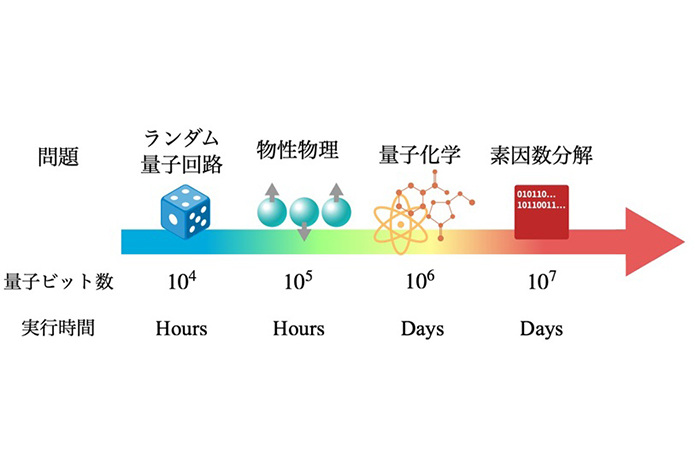